OXYGEN &
CARBON DIOXIDE TRANSPORT BY BLOOD
General
Goal To describe the storage of oxygen and carbon
dioxide in blood and tissues, and to provide a framework for understanding how
these stores interact.
Specific
Objectives The student should:
be able to name the major regions of
drop as blood moves
from alveolus to mitochondria.
know the shape of the myoglobin
dissociation curve, and be able to state the two major functions of myoglobin.
know what is meant by the P50
of hemoglobin, be able to sketch a normal oxygen dissociation curve, and be
able to state the significance of the flat portion for oxygen loading, and the
steep portion for oxygen unloading.
be able to define oxygen carrying
capacity, oxygen content, oxygen saturation, and oxygen partial pressure.
be able to describe the effects of pH,
2,3-DPG, temperature
, and
on hemoglobin P50.
be able to describe the effects of
methemoglobinemia, carboxyhemoglobin, and anemia on oxygen carrying capacity.
be able to define the Bohr effect, the
Haldane effect, and the chloride shift.
be able to quantitate the way in which CO2
is carried in plasma and the red cells.
be able to describe the significant
differences between the oxygen dissociation curve and the carbon dioxide
dissociation curve.
Resources Lecture: Dr. Baer
Reading: West, JB. Respiratory Physiology-The Essentials (4th
Ed.). Chapter 6.
Mines,
AH. Respiratory Physiology. Raven Press. Chapters 4, 5, & 6.
I.
OXYGEN
TENSIONS FROM ALVEOLI TO MITOCHONDRIA
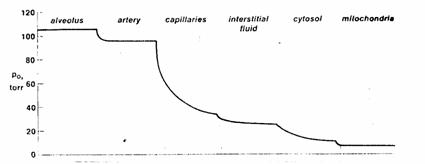
Figure 1. The transport of oxygen in higher organisms
requires the presence of concentration gradients at several sites, with the
oxygen tension progressively falling from the lung alveoli to the mitochondria
in the peripheral tissues where oxygen is consumed.
A.
Although
oxygen tension falls in several places, the greatest fall in oxygen tension
occurs across the systemic capillaries.
B.
Mixed
venous
is normally 40 mmHg.
Some
tissues extract a greater proportion of oxygen from arterial blood and have a
.
Some
tissues extract a lesser proportion of the oxygen from arterial blood and have
a
.
C.
The
partial pressure of oxygen in the mitochondria is often considerably lower than
the
at the end of the
systemic capillary. It is therefore
unfair to use the
of an organ as a
measure of its cellular
.
D.
Diffusion-limited transport. The existence of an end-capillary gradient
in some peripheral
tissues is indicative of diffusion limited transport.
E.
The
total body oxygen stores include:
oxygen
in the cells.
oxygen
in the blood.
oxygen
in the lung.
II.
CELLULAR
OXYGEN STORES AND MYOGLOBIN
A.
Cells
without myoglobin have oxygen stores limited by oxygens solubility.
B.
Myoglobin
is a protein which binds oxygen in red muscle. Red muscle includes the heart which is a continuously working aerobic
organ and red skeletal muscle used in the performance of aerobic or endurance
exercise.
C.
Myoglobin
Functions. There are 2 probable
functions.
Buffer
changes in tissue
during changes in
cellular metabolism.
Facilitate
O2 diffusion through the cytoplasm to the mitochondria where the O2
is used.
D.
Myoglobin
dissociation curve.
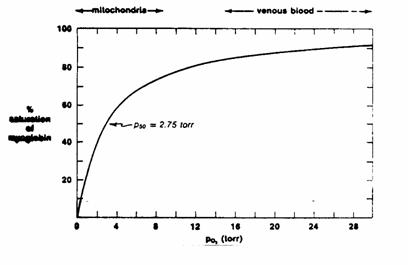
Figure 2
Myoglobin
is a single polypeptide chain containing 1 heme group. Each molecule of myoglobin binds one molecule
of O2.
Myoglobin
binding kinetics obey simple mass action laws and are therefore
Michaelis-Menton kinetics.
The
P50 of myoglobin is the partial pressure at which 50% of the
myoglobin molecules (or 50% of the myoglobin binding sites) have bound O2. This is by analogy to the Km of an
enzyme.
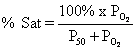
At
venous
levels, myoglobin is
nearly fully saturated.
At
mitochondrial
levels, a small fall
in
causes myoglobin to
release most of its oxygen for use (steep part of curve).
E.
Myoglobin
is also thought to facilitate intracellular O2 transport.
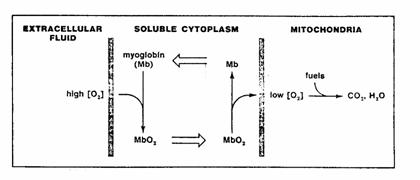
Figure 3. The presence of myoglobin can facilitate
diffusion within a cell. If the
concentration gradient of O2 is in a range over which the
concentration of oxygen greatly affects the degree of its binding to myoglobin,
much more of the myoglobin will be oxygenated on the high side of the
gradient. Effective concentration
gradients will therefore be established for oxymyoglobin (MbO2) in
one direction and for deoxymyoglobin (Mb) in the opposite direction, so that
molecules of the carrier will go to and fro across the concentration gradient,
picking up O2 on the high side, and releasing it on the low.
III.
BLOOD
OXYGEN STORES AND HEMOGLOBIN
A.
Oxygen
is carried in blood in 2 forms
Dissolved
in plasma. Normally insignificant.
Bound
to hemoglobin in red blood cells.
B.
Ways
of expressing the amount of oxygen in blood.
O2 Partial Pressure. (Also called oxygen tension.) Units are mmHg. The partial
pressure of oxygen is proportional to the amount of O2 dissolved
in blood. Partial pressure differences
are of significance in determining the rate of diffusion between blood and
tissue.
O2 Content. Units are ml O2/dl blood. These are often written ml/dl. An
older synonym is Vol%. By convention
volume units are substituted for molar units (moles/liter) although the later
could be calculated by the gas law. O2
content is the total amount of oxygen in a deciliter of blood and includes both
dissolved and hemoglobin-bound oxygen, but dissolved [Hb] is normally
negligable. Ignoring dissolved Hb:


O2 Saturation. This is the fraction or percentage of all the hemoglobin binding sites
that are currently occupied by oxygen. Units are usually percent.

Since dissolved
O2 content is normally negligible, saturation can be thought of as
content/capacity.
C.
Dissolved O2. The arterial plasma normally contains:

D.
Hemoglobin
contains 2 alpha chains and 2 beta chains each of which contains 1 heme
group. One molecule of hemoglobin can
bind 4 molecules of O2.
One
g of hemoglobin can bind 1.36 ml O2. (The numbers 1.34 ml O2 and 1.39 ml O2 are also in
common use.)
Blood
normally contains 15 g/dl of hemoglobin.
From
above, the oxygen binding capacity of blood with a normal hemoglobin is:

E.
When
breathing 100% O2
dissolved O2
contributes 1.8 ml O2/dl blood.
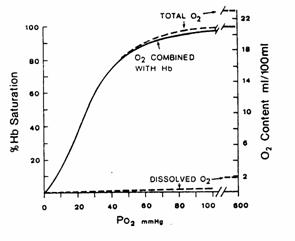
Figure 4. O2 dissociation curve (solid line)
for pH 7.4 PCO2 40 mmHg and
37C. The total blood O2
content is also shown for a hemoglobin concentration of 15 g/100 ml of blood.
F.
Sigmoid binding curve. Cooperativity causes the oxygen dissociation curve to display sigmoid
kinetics.
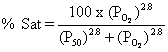
The
Hill coefficient is 2.8 (not the expected 4) because cooperativity is not complete.
The
P50 for hemoglobin (half the binding sites saturated) is 27 mmHg as
opposed to 2.8 mmHg for myoglobin.
If
there were no cooperativity (Hill coefficient of 1) and a P50 = 27
mmHg, then hemoglobin would take up less O2 at the lungs (only 79%
saturated at
) and would give up less O2 as
fell to venous levels.
G.
Significance
of the Sigmoid curve
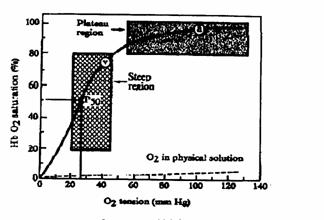
Figure 5. Oxygen-hemoglobin dissociation curve. The driving pressure for loading O2
onto Hb is arterial O2 tension, which is represented by the amount
of O2 physically dissolved in solution: a = arterial; v = venous; P50
= O2 tension required to saturate 50% of the hemoglobin.
Hemoglobin
is 90% saturated even when arterial
falls to 60 mmHg. This is because the upper portion of the
curve is relatively flat.
In
systemic capillaries where blood
may be low
hemoglobin becomes
desaturated, i.e., it releases oxygen for uptake by cells. This is the steep portion of the curve.
Normally
hemoglobin gives up less than half its O2. There is reserve in the system. If systemic capillary
falls further (eg. in
exercise) there is plenty of additional O2 available from
hemoglobin.
H.
Tabular
oxygen dissociation curve (*remember these to sketch your own)
*Values needed reasonably
accurate dissociation curve. Remember
that the PO2 at which the saturation is 50% (the P50, detailed
later) 27 mmHg.
|
Blood 
|
%O2 Saturation
|
|
|
|
|
|
|
|
|
|
|
|
|
|
|
|
|
|
|
|
|
|
|
IV.
CHANGES
IN HEMOGLOBIN AFFINITY FOR OXYGEN
A.
Changes
in hemoglobin affinity for oxygen result in a change in P50. Note that the maximum oxygen capacity is not
changed. (change in Km not
Vmax.)
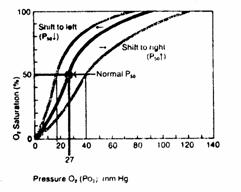
Figure 6
Increased hemoglobin affinity for O2. The oxygen dissociation curve shifts to the left. P50 is reduced.
Decreased hemoglobin affinity for O2. The oxygen dissociation curve shifts to the right. P50 is increased.
B.
Shifts
in oxyhemoglobin dissociation curve have minimal effects on oxygen loading in
the lung because the oxygen dissociation curve is still fairly flat at a
of 100 mmHg.
C.
Shifts
in the oxyhemoglobin dissociation curve have a maximal effect on oxygen
unloading at the tissues because the curve is steep at venous
(eg. 40 mmHg).
D.
Hydrogen Ion. Increased [H+] (decreased pH) increases the P50
(decreased O2 affinity) and shifts the dissociation curve to the
right. Decreasing [H+] the
opposite effect.
Figure 7
Acidosis promotes oxygen unloading. At
compare
at pH = 7.4 with
at pH = 7.2.
Alkalosis
inhibits oxygen unloading.
E.
Carbon dioxide
(Bohr Effect). Increasing
increases P50. Decreasing
decreases P
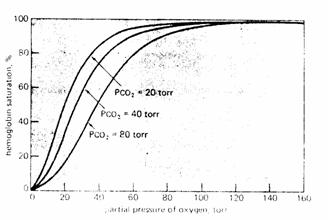
Figure 8
The major portion of the Bohr Effect is due to the fact
that increasing
causes a decreased red cell pH
(acidosis).
A secondary
part of the Bohr Effect is due to the fact that CO2 reacts
covalently with hemoglobin to form carbamino hemoglobin which has a reduced O2
affinity.

The
Bohr Effect plays a significant role in oxygen unloading under normal,
physiological conditions. The P50
of a venous oxygen dissociation curve may be as high as 35 mmHg as compared
to the normal arterial value of 27 mmHg.
F.
Temperature. Increasing temperature increases P50. Decreasing temperature decreases P50.
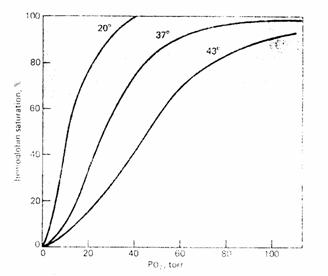
Figure 9
G.
Exercise. Increase temperature, increased
and decreased pH all promote oxygen
unloading at the tissues by increasing P50 (decreased affinity).
H.
2,3-diphosphoglycerate (2,3-DPG). 2,3-DPG is a glycolytic intermediate which accumulates to uniquely high
levels in red cells. Increased levels
increase P50; decreased levels decrease P50. Increased levels occur in association with
hypoxia.
Increased
levels of 2,3-DPG may be seen in:
a)
acclimatization
to high altitudes.
b)
chronic
lung disease; emphysema.
c)
anemia.
d)
hyperthyroidism.
e)
right
to left cardiac shunt.
f)
congenital
heart disease.
g)
pulmonary
vascular disease.
Blood bank storage. Blood storage in citrate-phosphate-dextrose solution for as short as 1
week can lead to significant 2,3-DPG depletion and left-shifted oxygen
dissociation curves.
Fetal hemoglobin. 2,3-DPG levels do not affect the P50 of fetal hemoglobin.
V.
CHANGES
IN O2 CARRYING CAPACITY OF HEMOGLOBIN
A.
Hemoglobin
Concentration. Hemoglobin concentration
will change from its normal value of 15 g/dl as hematocrit changes.
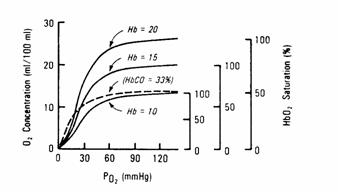
Figure 10
Anemia will decrease the oxygen carrying capacity of blood without
independently altering the P50 of blood.
Polycythemia will increase the oxygen carrying
capacity of blood without independently altering the P50 of blood.
B.
Carbon Monoxide. Carbon monoxide binds hemoglobin at the oxygen binding sites to form
carboxyhemoglobin. It has approximately
210 times the affinity of oxygen.
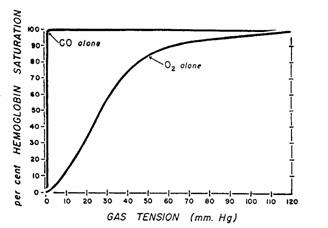
Figure 11.Dissociation curves for HbO2
and HbCO. Dissociation curves are plotted
on the same scale. Maximal saturation of
hemoglobin with O2 is not reached until the PO2 is
greater than 120 mmHg; with CO, however, maximal saturation is attained with PCO
of less than 1 mmHg.
Occupation
of binding sites by CO effectively eliminates them for O2 binding
and therefore reduces O2 binding capacity (see figure in Section A
above).
Because
of the complex cooperativity between hemoglobin subunits, binding of some sites
by CO also reduces P50 for oxygen binding. Note that this does not occur with anemia.
C.
Methemoglobin. The heme groups of hemoglobin normally contain ferrous iron (Fe++)
whether bound to oxygen or not.
Oxidizing Agents. Certain drugs and chemicals (eg. nitrates, acetanilide, sulfonamides)
can oxidize the heme Fe++ to Fe+++. The resulting hemoglobin is called
methemoglobin and does not bind O2. The total oxygen carrying capacity is reduced by the amount of
hemoglobin that is methemoglobin.
A
certain amount of methemoglobin forms spontaneously. This is normally less than 1% of total
hemoglobin. The enzyme
NADH-methemoglobin reductase helps prevent accumulation of methemoglobin.
VI.
CARBON
DIOXIDE STORES
A.
The
solubility of carbon dioxide is 0.075 ml CO2/dl/mmHg.
B.
Carbon
dioxide is carried in the plasma in two forms.
Dissolved CO2. At
the dissolved CO2
concentration is 3.4 ml/dl.
Carbamino compounds. Plasma protein concentration is about 7%. CO2 binds the amine groups of
plasma proteins to form carbamino compounds. The hydrogen ions formed are buffered by plasma proteins.


Plasma
has little carbonic anhydrase so CO2 forms little carbonic acid in
plasma.
C.
Carbon
dioxide is carried by the red blood cell in three forms.
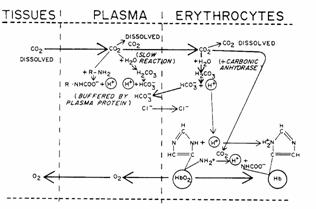
Figure 12
Dissolved CO2. CO2 can cross the red cell membrane and dissolve in RBC
water.
Carbamino compounds. Approximately 30% of RBC contents is hemoglobin. CO2 can form carbamino hemoglobin
on amine groups. The H+
released by this reaction is buffered by histidine residues (imidazole group) on
the hemoglobin itself.
Bicarbonate. Carbonic anhydrase is present in RBCs and catalyze the formation of
carbonic acid which dissociated to hydrogen ion and bicarbonate. The H+ is buffered by hemoglobin.

D.
Chloride Shift. As
is formed it diffuses out of the red cell. Cl- diffuses into the red
cell to maintain electroneutrality. This
is the Chloride Shift or Hamburger Shift.
The
chloride shift is rapid and is complete before the cells exit the capillary.
The
osmotic effect of the extra
and Cl- in venous red cells
causes the venous RBC volume to increase slightly. For this reason venous hematocrit slightly
exceeds arterial hematocrit.
E.
Quantitative
summary of CO2 transport.
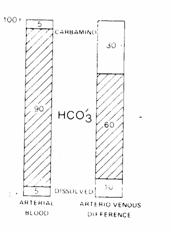
Figure 13
90% of arterial CO2 stores are carried as
with 5% of the stores carried as
dissolved CO , and 5% of the stores as carbamino compounds.
Of the CO2 added in systemic capillaries, 60%
is added as
, 30% is added as carbamino compounds
and 10% is added as dissolved CO .
VII.
CARBON
DIOXIDE DISSOCIATION CURVE
A.
The
total CO2 content of blood can be plotted as a function of
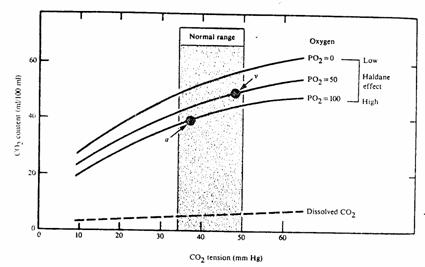
Figure 14
B.
Haldane effect. Increasing O2 tension decreases the affinity of hemoglobin
for CO2. As a result the CO2
dissociation curve shifts downward.
High
promotes CO2 unloading in the
lungs.
Low
promotes CO2 loading in the
periphery.
C.
Comparison
of CO2 and O2 dissociation curves.
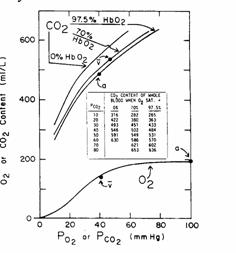
Figure 15
CO2
content is far higher than O2 content at physiological partial
pressures.
The
CO2 dissociation curve is approximately linear (a straight line) in
the range of arterial and venous blood. Thus, if equal volumes of blood of 2 different CO2 contents
are mixed together, the resulting
will be half way between the starting
values.
The
O2 dissociation is curved between arterial and venous points. Thus, if equal volumes of blood of 2
different O2 contents are mixed together, the resulting blood will
have a
which is not centered between the original
values.
VIII.
CARBON
DIOXIDE AND ACID-BASE BALANCE
A.
The
lungs excrete 13,000 mEq a day of CO2 representing an equivalent
volume of carbonic acid. The kidneys
excrete 40-80 mEq/day fixed acid.
B.
Henderson-Hasselbach
Equation

C.
Changes in
cause changes in [H+]
by mass action.
An increase in
results in respiratory acidosis.
An decrease in
results in respiratory alkalosis.
D.
The effect of altered
on pH depends on whether the bicarbonate
buffer system acts alone or in concert with other buffer systems.
TABLE 1. Effects of adding CO2 to fluids
with and without buffers other than bicarbonate
Type of Fluid
|

(mEq/liter)
|
pH
|
[H+]
(nEq/liter)
|
(mmHg)
|
|
A. Fluid with no nonbicarbonate buffers of
importance (CSF)
|
|
|
|
|
INITIAL
FINAL
|
B. Fluid with buffers in addition to
bicarbonate (blood)
|
|
|
|
|
INITIAL
FINAL
|
CSF. The bicarbonate buffer system works alone and
has a larger effect on pH.
Blood. Hemoglobin buffers H+ changes in
addition to
. Thus, changes in
have somewhat blunted effect on pH.
STUDY
QUESTIONS FOR OXYGEN AND CARBON DIOXIDE TRANSPORT
What is meant by the term P50?
What is the Bohr Effect? The Haldane Effect?
List four factors which will increase the
P50 for hemoglobin. What is
the functional significance of an increase P50 (rightward shift in
the O2 dissociation curve)?
How will each of the following affect
and oxygen content of
arterial blood
? a) Anemia; b) Polycythemia; c) Low
in inspired air; d)
2,3 DPG; e) Increased
; f) Fever; g) Carbon
monoxide poisoning; h) Methemoglobinemia
What effect does oxygen uptake in the
lungs have on the buffering capacity of blood? What does oxygen unloading in tissues have?
How does oxygen unloading in tissues
affect carbon dioxide uptake by blood?
How does oxygen loading in lungs affect
carbon dioxide release from blood?
What role does the chloride shift play in
carbon dioxide transport by blood?
In what form is carbon dioxide carried in
blood? What is the percent contribution
of each?
Calculate the oxygen carrying capacity of
a person with hemoglobin concentration of 18 g/dl. What is his approximate arterial saturation
if his
is 17 ml/dl?
In a normal person,
what would be the O2 saturation at a
of 40 mmHg? At 27 mmHg? At 60 mmHg?
Of what significance is the fact that the
CO2 dissociation curve is linear over the normal range? Hint: how does this affect mixing of blood?